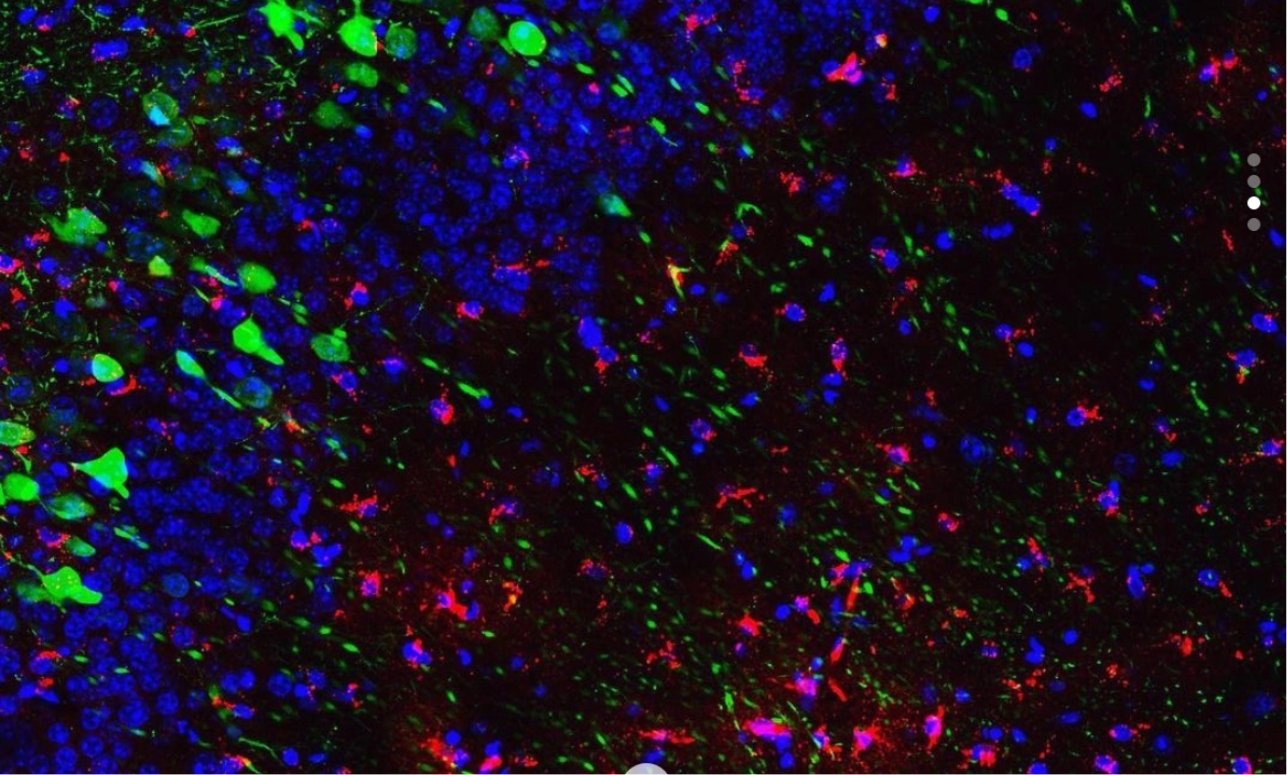
Research
Hidden between your ears is the one thing that should give physicists neuroscience-envy: a time machine seamlessly capable of transporting you back to a memory of your first kiss, the time you got into college, or the Malcom Butler interception that won the Patriots the Super Bowl in 2015.
In the lab, we will break into this time machine, reverse engineer it and, ultimately, control it. It’s not a matter of if, it’s a matter of when and how.
Our Mission
The mission of our lab is twofold: to reveal the neural circuit mechanisms of memory storage and retrieval, and to artificially modulate memories to combat mental health disorders. We will do so in a multi-disciplinary fashion by combining virus engineering strategies, immunohistochemistry, physiology, optogenetics, functional imaging of targeted populations in vivo, and a battery of behavioral assays. Our technical repertoire, of course, will evolve as our studies evolve.
Given that any given brain region interacts with numerous targets along exquisitely precise spatial and temporal dimensions — nothing in the brain exists in a vacuum — we’ll study the circuit-wide and behavioral manifestations of learning and memory across a variety of structurally and functionally connected areas. We believe that a systems-level analysis of memory that examines genetically-defined projection-specific cell-types, while simultaneously surveying their real-time physiological dynamics, is a tractable experimental path towards understanding and controlling this complex, ephemeral process.
Selected Public Outreach
The neuronal mechanisms of learning and memory
Previous studies have suggested that a sparse population of neurons distributed throughout the brain encodes a specific memory. These neurons can be tagged during learning for subsequent identification and manipulation. In particular, the hippocampus and its cortical connections are pivotal for the encoding, storage, retrieval, and updating of episodic memories. Previously, our work has demonstrated that directly re-activating hippocampus cells that were active during learning was sufficient to recreate the neuronal and behavioral expression of the previously learned memory.
One line of experiments focuses on identifying cells in the hippocampus that are active during learning to explore the brain pathways and processes that give rise to memory. This includes studying how the hippocampus communicates to various other brain areas during the recollection of an experience, as well as to study the molecular and physiological identity of these “memory-bearing cells.”
Another line of experiments focuses on chronically manipulating memory-bearing cells in the brain to lastingly reprogram neuronal circuits and behaviors, as well as characterizing the underlying neuronal landscape in vivo.
For these basic science projects, our conceptual scaffold consists of asking “who talks to whom and when,” thereby permitting us to probe the putative neuronal processes underpinning learning and memory. These projects also begin to guide ongoing and future experiments which combine the fields of learning and memory with animal models of psychiatric disorders.
Manipulating memories to combat psychiatric disorders
Chronic stress affects numerous brain areas involved in memory, emotion, and motivation— such as the hippocampus and various cortical areas. In these areas, stress abnormally alters a variety of cellular events and can precipitate several maladaptive states, such as depression, anxiety, and post-traumatic stress disorder (PTSD). Yet, the neural circuitry or processes that are able to mitigate or prevent such pathological phenotypes is unclear, and most interventions rely on historically ineffective drug-based strategies.
A tractable path forward includes at least two key conceptual and experimental shifts:
Deconstructing pathologies into symptom clusters with shared neurophysiological, anatomical, and biochemical substrates in humans to provide putative nodes for interrogation in rodents.
A shift in the view that a given brain region, transmitter, or modulator subserves a particular cognitive function to a more nuanced view focusing on the real-time activity and topology of genetically-defined, projection-, target-, and synapse-specific neural networks interacting during adaptive and maladaptive states.
To begin to implement these shifts in approach — and given the extraordinary evolutionary conservation of the structural and functional properties of cortico-hippocampus memory networks — we will commandeer the brain’s endogenous plasticity mechanisms by means of artificial memory modulation to test for and resolve its potential to regulate maladaptive circuit functioning and behavior. This proposed research aims to provide a neurobiological framework for artificially activating memories to bi-directionally modulate stress-induced states.
The second line of experiments seeks to leverage our understanding of the endogenous cellular processes supporting the various stages of memory (e.g. (re)consolidation, extinction) in the hippocampus-amygdala-prefrontal cortex pathway to intervene with disorders of fear regulation (e.g. PTSD).
The goal of these experiments is to provide the biomedical community with neuronal, physiological, and behavioral markers that can be leveraged to develop interventional and preventative therapies to ameliorate the maladaptive states that define many neuropsychiatric disorders.